Key Concepts in Physics
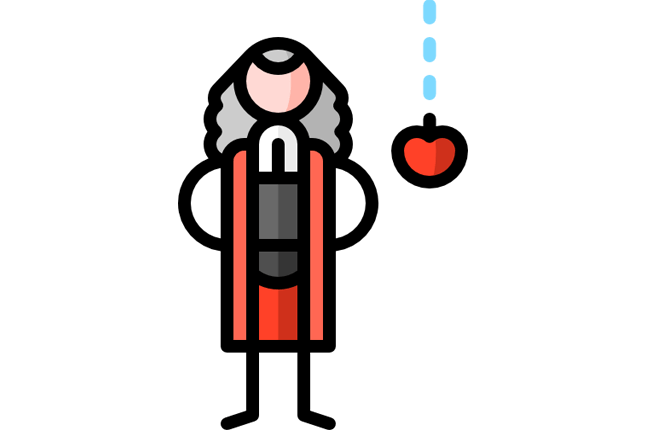
Newtonian Mechanics
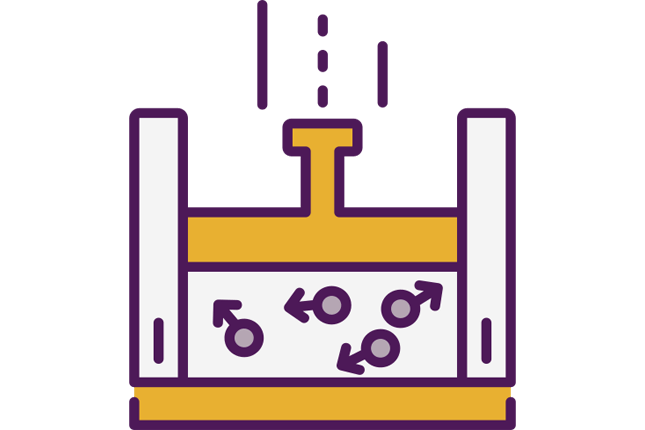
Thermodynamics
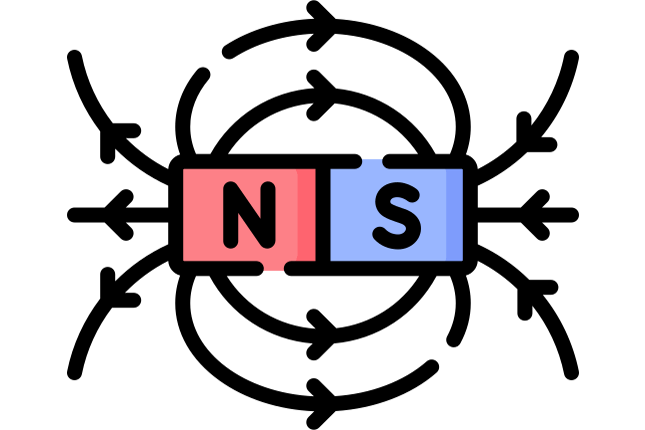
Electricity & Magnetism
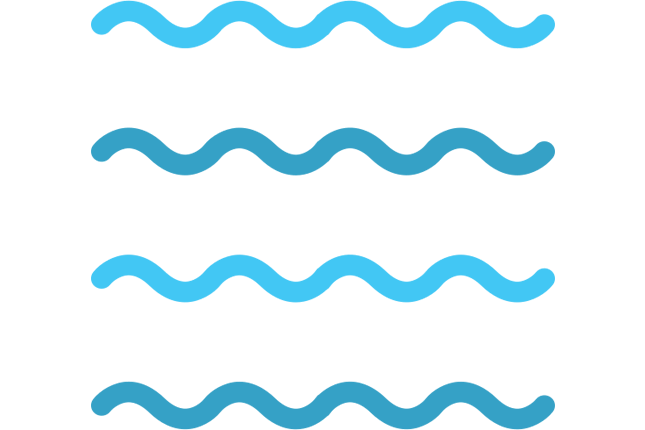
Waves & Optics
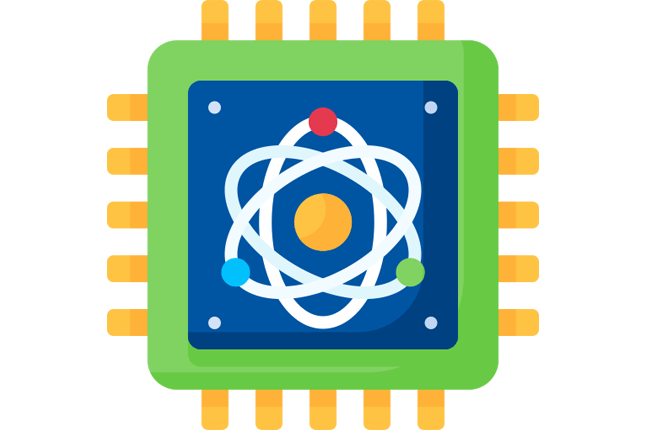
Modern Physics
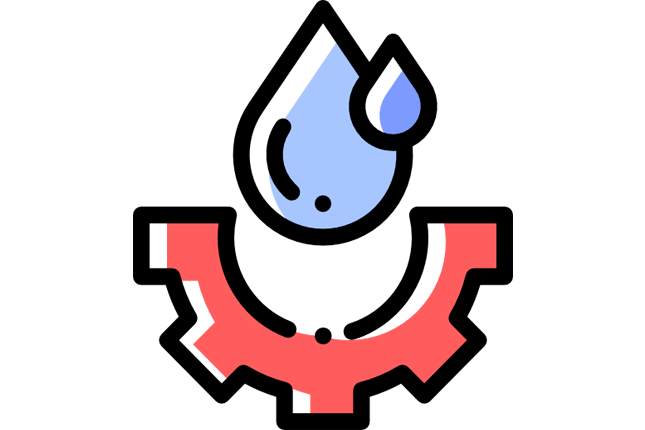
Fluid Mechanics
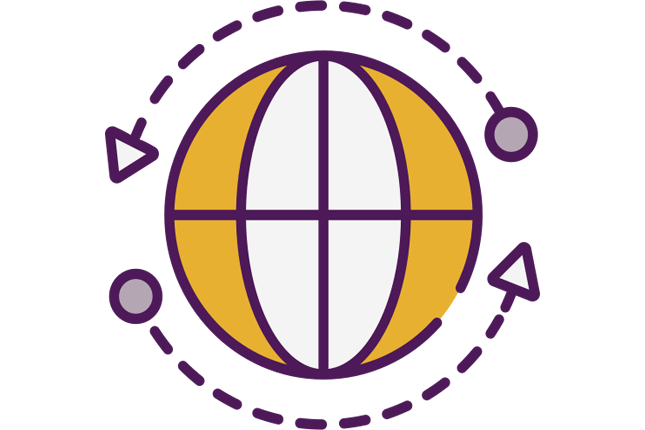
Circular & Rotational Motion
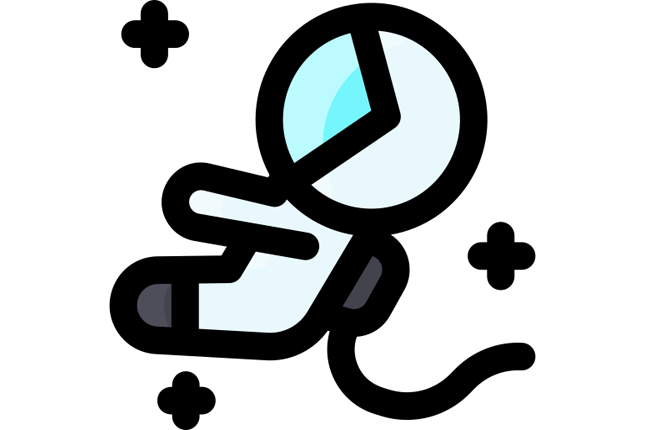
Gravitation
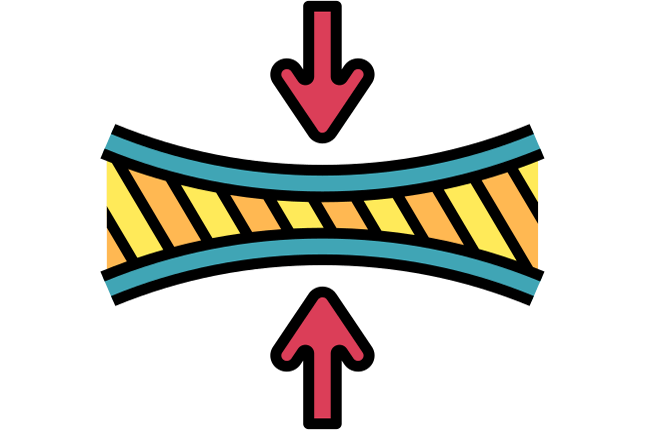
Materials & Properties
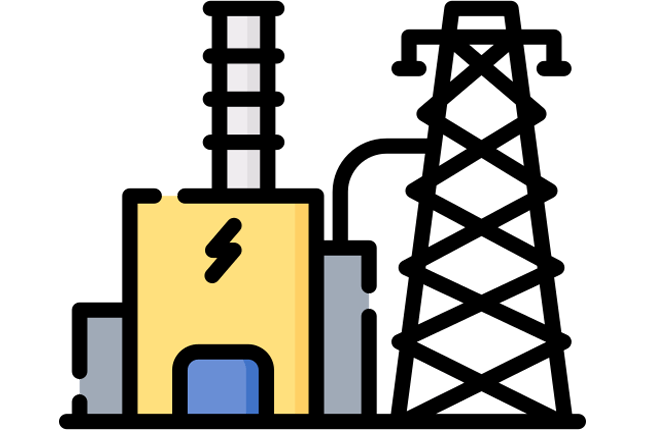
Energy & Power
Physics Essentials – Concepts and Practical Examples
Physics is the fundamental science that explores the laws governing the natural world. It provides a framework for understanding the forces, energy, and matter that shape our universe. This comprehensive guide covers essential physics topics and their practical applications, offering detailed explanations and real-world examples.
Newtonian Mechanics
Newtonian mechanics, developed by Sir Isaac Newton, forms the foundation of classical physics. It describes the motion of objects and the forces acting upon them.
Laws of Motion
Newton’s three laws of motion explain how objects move and interact. The first law, also known as the law of inertia, states that an object at rest stays at rest, and an object in motion stays in motion unless acted upon by an external force.
The second law relates force, mass, and acceleration, providing the equation
The third law states that for every action, there is an equal and opposite reaction.
In everyday life, these laws explain everything from how cars accelerate and brake to why we feel a pushback when we push against a wall.
Kinematics and Dynamics
Kinematics studies the motion of objects without considering the forces causing the motion. It involves concepts such as velocity, acceleration, and displacement. Dynamics, on the other hand, focuses on the forces and their effects on motion.
For instance, calculating the trajectory of a projectile, like a football, involves kinematic equations. Engineers use dynamics to design safe and efficient transportation systems by understanding how forces impact vehicle motion.
Circular Motion and Centripetal Force
Objects moving in a circular path experience centripetal force, which is directed towards the center of the circle. This force is crucial for understanding phenomena such as the orbits of planets and the operation of centrifuges in laboratories.
In amusement parks, roller coasters are designed using principles of circular motion to ensure that the centripetal forces keep the cars on the track during loops and turns.
Work, Energy, and Power
Work is done when a force moves an object over a distance. Energy is the capacity to do work, and power is the rate at which work is done. The principle of conservation of energy states that energy cannot be created or destroyed, only transformed from one form to another.
In real-world applications, understanding these concepts is essential for everything from designing engines and power plants to developing sustainable energy solutions.
Conservation of Momentum
The conservation of momentum principle states that the total momentum of a closed system remains constant unless acted upon by external forces. This concept is critical in analyzing collisions and interactions between objects.
In sports, for example, understanding momentum helps in improving techniques in games like soccer or basketball, where controlling the ball’s momentum is key to successful play.
Thermodynamics
Thermodynamics studies the relationships between heat, work, temperature, and energy. It has profound implications for various industries, including automotive, aerospace, and environmental science.
Laws of Thermodynamics
The first law of thermodynamics, also known as the law of energy conservation, states that energy cannot be created or destroyed. The second law introduces the concept of entropy, indicating that energy tends to spread out or disperse, leading to an increase in disorder. The third law states that as the temperature approaches absolute zero, the entropy of a system approaches a constant minimum.
These laws are fundamental in understanding how engines work, how refrigerators cool, and why perpetual motion machines are impossible.
Heat Transfer
Heat can be transferred through conduction, convection, and radiation. Conduction occurs through direct contact, convection through fluid movement, and radiation through electromagnetic waves.
In everyday life, heat transfer principles explain why metal spoons get hot when left in a pot of boiling water (conduction), how heaters warm up a room (convection), and how the sun warms the Earth (radiation).
Thermal Expansion
Materials expand when heated and contract when cooled. This property is considered in construction and manufacturing to prevent structural damage due to temperature changes.
Bridges, for example, have expansion joints to accommodate the expansion and contraction of materials, ensuring structural integrity throughout seasonal temperature variations.
Entropy and the Second Law of Thermodynamics
Entropy measures the disorder or randomness of a system. The second law of thermodynamics states that in any energy transfer, the total entropy of a system and its surroundings always increases.
This principle explains why natural processes tend to move towards a state of disorder, such as why ice melts in a warm room or why buildings and machines wear down over time.
Electricity and Magnetism
Electricity and magnetism are closely related phenomena that play a vital role in modern technology.
Electric Charge and Electric Field
Electric charge is a fundamental property of matter that causes it to experience a force when placed in an electric field. Positive and negative charges attract each other, while like charges repel.
In practical terms, electric charges and fields are harnessed in capacitors, which store energy in electronic circuits, and in electrostatic applications like photocopiers and air purifiers.
Electric Potential and Voltage
Electric potential is the energy per unit charge at a point in an electric field, and voltage is the difference in electric potential between two points.
Voltage is a critical concept in electrical engineering, determining the operation of batteries, power supplies, and electrical grids.
Current, Resistance, and Ohm’s Law
Electric current is the flow of electric charge, resistance is the opposition to this flow, and Ohm’s law relates the two through the equation .
Understanding these concepts is crucial for designing electrical circuits and components, from household appliances to advanced computing systems.
Series and Parallel Circuits
In series circuits, components are connected end-to-end, so the same current flows through each. In parallel circuits, components are connected across common points, allowing multiple paths for the current.
Knowing how to design and analyze series and parallel circuits is essential for electricians and engineers working with complex electrical systems.
Magnetic Fields and Electromagnetism
Magnetic fields are generated by moving electric charges, and electromagnetism describes the interaction between electric currents and magnetic fields.
Applications of electromagnetism include electric motors, transformers, and MRI machines, which rely on the principles of magnetic fields and induction.
Faraday’s Law of Induction
Faraday’s law states that a changing magnetic field induces an electric current in a conductor. This principle is the basis for electric generators and transformers.
Wind turbines and hydroelectric dams use Faraday’s law to convert kinetic energy into electrical energy, providing renewable power sources.
Alternating Current (AC) and Direct Current (DC)
AC and DC describe the flow of electric charge. AC alternates direction periodically, while DC flows in one direction.
Most household appliances use AC power, while batteries and electronic devices typically use DC power. Understanding both types is essential for designing and maintaining electrical systems.
Waves and Optics
Waves and optics study the behavior of waves and light, fundamental to numerous technologies.
Properties of Waves
Waves are characterized by properties such as frequency, wavelength, and amplitude. These properties determine the energy and behavior of waves.
Understanding wave properties is crucial for designing communication systems, from radio and television broadcasting to wireless internet and cellular networks.
Sound Waves and Acoustics
Sound waves are mechanical waves that travel through a medium, and acoustics is the study of sound.
Acoustics is applied in designing concert halls for optimal sound quality, in noise reduction for buildings and vehicles, and in medical diagnostics with ultrasound technology.
Light Waves and the Electromagnetic Spectrum
Light waves are part of the electromagnetic spectrum, which includes radio waves, microwaves, infrared, visible light, ultraviolet, X-rays, and gamma rays.
Understanding the electromagnetic spectrum is essential for technologies like satellite communication, medical imaging, and even everyday items like microwave ovens and remote controls.
Reflection, Refraction, and Diffraction
Light can reflect off surfaces, bend when passing through different media (refraction), and spread out when passing through small openings (diffraction).
These principles are fundamental in designing lenses for glasses and cameras, fiber optic cables for telecommunications, and various optical instruments.
Lenses and Mirrors
Lenses and mirrors manipulate light to form images. Convex and concave lenses focus and diverge light, respectively, while mirrors reflect light.
Optical devices like microscopes, telescopes, and cameras rely on the principles of lenses and mirrors to magnify and capture images of distant or tiny objects.
Optical Instruments
Optical instruments such as microscopes and telescopes extend our ability to observe the microscopic and astronomical worlds.
Microscopes are essential in biology and medicine for studying cells and tissues, while telescopes allow astronomers to explore the universe, from observing planets and stars to discovering distant galaxies.
Modern Physics
Modern physics explores phenomena beyond classical mechanics, delving into the realms of relativity and quantum mechanics.
Relativity
Albert Einstein’s theories of relativity revolutionized our understanding of space, time, and gravity. Special relativity deals with objects moving at high speeds, while general relativity explains gravity as the curvature of spacetime.
Relativity has practical applications in technologies like GPS, which requires precise time measurements to provide accurate positioning data.
Quantum Mechanics
Quantum mechanics describes the behavior of particles at the atomic and subatomic levels, where classical physics fails. It introduces concepts such as wave-particle duality and quantum entanglement.
Quantum mechanics underpins modern technologies like transistors, lasers, and quantum computing, which promise to revolutionize data processing and encryption.
Atomic Models and Spectroscopy
Atomic models explain the structure of atoms, while spectroscopy analyzes the interaction of light with matter to determine the composition of substances.
Spectroscopy is widely used in chemical analysis, environmental monitoring, and even astronomy, where it helps identify the elements present in stars and galaxies.
Nuclear Physics
Nuclear physics studies the components and behavior of atomic nuclei. It includes phenomena like radioactivity, nuclear fission, and fusion.
Nuclear physics has applications in energy production, with nuclear power plants providing a significant portion of the world’s electricity, and in medicine, where nuclear technology is used for imaging and cancer treatment through radiation therapy.
Particle Physics
Particle physics explores the fundamental particles that make up the universe, such as quarks, leptons, and bosons. It also studies the forces that govern their interactions.
This field is critical for understanding the universe at its most basic level, and has practical applications in developing new technologies, including medical imaging devices like PET scans, and in advancing our understanding of the cosmos through experiments conducted in particle accelerators like the Large Hadron Collider.
Applications of Quantum Mechanics
Quantum mechanics is not just a theoretical discipline; it has profound real-world applications. Lasers, which are ubiquitous in modern technology, from barcode scanners to eye surgery, operate on principles derived from quantum mechanics. Semiconductors, the building blocks of all modern electronics, are also based on quantum principles, which allow us to manipulate the flow of electrons in devices like computers and smartphones.
Fluid Mechanics
Fluid mechanics studies the behavior of liquids and gases in motion and at rest. It is fundamental to numerous engineering applications.
Properties of Fluids
Fluids have unique properties such as density and pressure that influence their behavior. Understanding these properties is essential for designing systems that involve fluid flow, such as pipelines, water supply systems, and HVAC systems.
For example, Bernoulli’s principle, which relates pressure and velocity in a flowing fluid, is applied in designing airplane wings to create lift, allowing aircraft to fly.
Buoyancy and Archimedes’ Principle
Buoyancy is the force exerted by a fluid that opposes the weight of an object immersed in it. Archimedes’ principle states that the buoyant force on an object is equal to the weight of the fluid displaced by the object.
This principle is critical in designing ships and submarines, ensuring that they float and maintain stability in water. It also explains why objects feel lighter when submerged in water.
Fluid Dynamics
Fluid dynamics studies the flow of fluids and includes concepts like viscosity, laminar flow, and turbulent flow. Understanding fluid dynamics is crucial for designing efficient hydraulic systems, predicting weather patterns, and even in medical applications like blood flow analysis.
Hydraulic systems, which use pressurized fluids to generate power, are employed in machinery ranging from car brakes to heavy construction equipment. Aerodynamics, a branch of fluid dynamics, is essential for designing vehicles and aircraft that minimize drag and maximize fuel efficiency.
Circular and Rotational Motion
Circular and rotational motion deals with objects that rotate or move in circular paths.
Rotational Kinematics
Rotational kinematics describes the motion of objects rotating about an axis, involving angular velocity, angular acceleration, and angular displacement.
In practical terms, understanding rotational kinematics is crucial for designing and analyzing rotating machinery, such as turbines and engines, which are fundamental to industries like power generation and automotive engineering.
Torque and Angular Momentum
Torque is the force that causes an object to rotate, while angular momentum is the rotational equivalent of linear momentum. These concepts are vital for understanding the stability and motion of rotating objects.
In sports, for example, the concept of torque explains how a diver or gymnast spins faster by tucking their limbs close to their body, reducing their moment of inertia and increasing angular velocity.
Rotational Inertia
Rotational inertia, or moment of inertia, is the resistance of an object to changes in its rotational motion. It depends on the mass of the object and the distribution of that mass relative to the axis of rotation.
Engineers must consider rotational inertia when designing rotating components, such as flywheels in engines, to ensure they operate efficiently and safely.
Gravitation
Gravitation is the force of attraction between objects with mass, and it plays a crucial role in both terrestrial and celestial mechanics.
Newton’s Law of Universal Gravitation
Newton’s law of universal gravitation states that every mass attracts every other mass with a force proportional to the product of their masses and inversely proportional to the square of the distance between them.
This law explains not only why apples fall to the ground but also governs the motion of planets, moons, and satellites, making it fundamental to fields like astronomy and space exploration.
Gravitational Fields and Potential Energy
Gravitational fields represent the influence that a massive object exerts on space around it, affecting other masses. Gravitational potential energy is the energy an object has due to its position in a gravitational field.
These concepts are used in calculating the trajectories of spacecraft, understanding tides, and even in constructing tall buildings, where the potential energy of objects must be carefully managed to ensure structural stability.
Orbital Motion
Orbital motion refers to the movement of objects like planets, moons, and satellites around a central body due to gravitational attraction.
Understanding orbital mechanics is essential for launching and maintaining satellites, which are critical for global communications, weather forecasting, and navigation systems like GPS.
Materials and Properties
The study of materials and their properties is integral to physics and engineering, influencing everything from construction to technology development.
Elasticity and Hooke’s Law
Elasticity describes a material’s ability to return to its original shape after being deformed, and Hooke’s law states that the force needed to extend or compress a spring is proportional to the distance it is stretched or compressed.
These principles are crucial in designing materials and structures that can withstand forces without permanent deformation, such as springs, bridges, and buildings.
Stress and Strain
Stress is the force applied to a material, while strain is the material’s deformation in response to that force. The relationship between stress and strain helps engineers determine how materials will behave under different conditions.
Understanding stress and strain is vital for ensuring that structures, from skyscrapers to aircraft, can endure the forces they encounter without failing.
Properties of Materials
Materials have various properties, including conductivity, hardness, and ductility, which determine their suitability for different applications.
For example, the conductivity of materials is crucial in the design of electrical circuits, while hardness and ductility influence the choice of materials for tools, machinery, and construction.
Energy and Power
Energy and power are central concepts in physics, underpinning much of modern technology and industry.
Kinetic and Potential Energy
Kinetic energy is the energy of motion, while potential energy is the energy stored due to an object’s position or state.
These forms of energy are foundational to everything from roller coasters, which convert potential energy into kinetic energy as they descend, to hydroelectric dams, which generate electricity by converting the potential energy of water into kinetic energy as it flows through turbines.
Conservation of Energy
The conservation of energy principle states that energy cannot be created or destroyed, only converted from one form to another. This principle is key to understanding how energy is transferred and transformed in systems ranging from simple machines to complex power grids.
In renewable energy systems, such as solar panels, the conservation of energy principle helps us understand how sunlight is converted into electrical energy, providing a sustainable power source.
Power Generation and Energy Efficiency
Power is the rate at which energy is transferred or converted. Understanding power generation and energy efficiency is crucial for developing sustainable energy systems and reducing environmental impact.
Engineers and scientists work to improve the efficiency of power plants, electric vehicles, and appliances to ensure that we can meet our energy needs while minimizing waste and environmental damage.
Renewable Energy Sources
Renewable energy sources, such as solar, wind, and hydroelectric power, are becoming increasingly important as we seek to reduce our reliance on fossil fuels.
Physics plays a crucial role in developing and optimizing these technologies, from understanding the aerodynamics of wind turbines to maximizing the efficiency of solar cells.
Wrapping It Up
Physics is a vast and dynamic field that influences every aspect of our lives. From the fundamental laws of motion to the complex interactions of particles in quantum mechanics, physics provides the tools we need to understand and manipulate the natural world. By exploring these essential topics, we gain insights that drive technological innovation, improve our quality of life, and help us solve some of the most pressing challenges of our time. Through this comprehensive understanding of physics, we can continue to harness its principles to build a better, more sustainable future.