Environmental Chemistry – Sources, Impact, and Solutions
Environmental chemistry examines how chemical processes influence the air, water, soil, and living organisms on our planet. It addresses sources of pollutants, how they move through ecosystems, and how to reduce or prevent harm. Scientists in this field measure contaminants, study their interactions, and advise on managing emissions or cleaning up waste. This guide offers a thorough discussion of key concepts, such as how pollutants form, how they travel, and the methods used to assess and limit environmental damage.
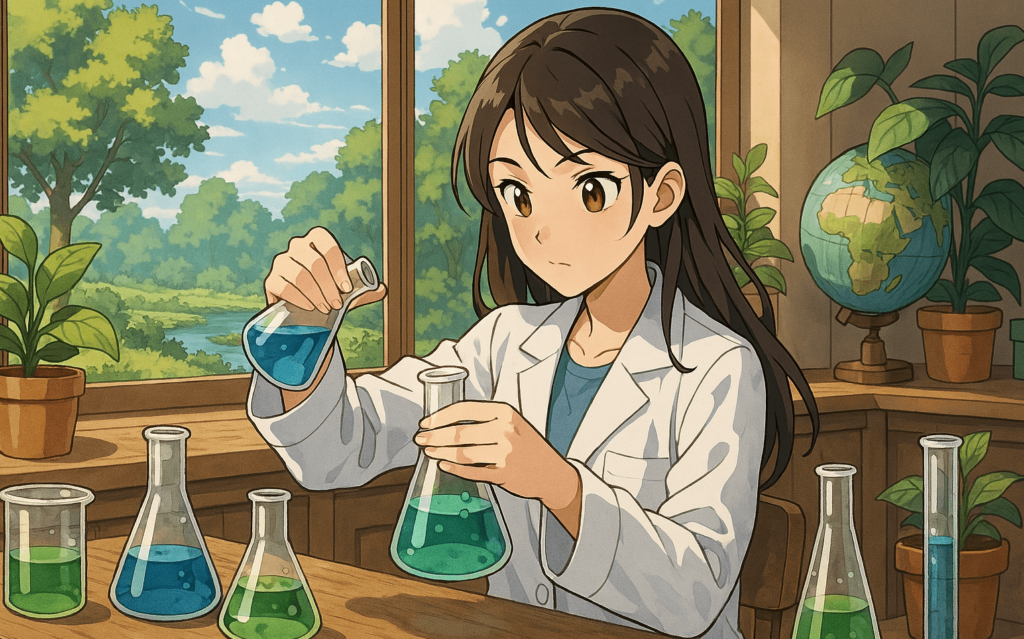
1. Introduction
Human activity changes the natural balance in air, rivers, oceans, and soils. Industries release byproducts, vehicles emit exhaust, and households produce waste. Even agriculture alters nutrient cycles in farmland and waterways. Environmental research seeks to clarify how these chemicals behave after release—where they settle, how toxic they might be, and what conditions might degrade them. Governments then formulate guidelines or laws to safeguard ecosystems and public health.
Environmental problems can seem overwhelming, spanning global challenges like climate change to local concerns such as leaking landfills. Each issue has a chemical aspect, from the breakdown of synthetic compounds to the rise in greenhouse gases. By understanding the underlying science, public policymakers and communities can make informed decisions about energy use, waste management, and resource protection.
2. Components of the Environment
2.1. Atmosphere
Air envelops Earth in layers of gases. The main components near ground level are nitrogen (around 78%) and oxygen (about 21%), along with small amounts of argon, carbon dioxide, and water vapor. Traces of pollutants like sulfur dioxide (SO₂), nitrogen oxides (NOₓ), particulate matter, and volatile organic compounds (VOCs) can harm health or trigger chemical reactions. The ozone layer in the stratosphere blocks harmful ultraviolet radiation, yet human-made substances (e.g., chlorofluorocarbons, or CFCs) weakened it in past decades, prompting global restrictions.
2.2. Hydrosphere
Water covers most of Earth, forming oceans, lakes, rivers, and underground aquifers. Water dissolves countless minerals and chemicals, which can lead to contamination. Pollutants like heavy metals (lead, mercury) or organic compounds (pesticides, industrial solvents) might accumulate in aquatic environments. Marine ecosystems can suffer from oil spills, nutrient overload (eutrophication), or plastic debris, affecting the seafood supply and entire food webs.
2.3. Lithosphere (Soil and Rocks)
Soil hosts microbes and plant roots, cycling elements like carbon, nitrogen, and phosphorus. Industrial spills or agricultural runoff can deposit dangerous chemicals in soils. Some substances bind strongly to mineral particles, while others leach into groundwater or volatilize. Soil contamination also affects nutrient availability, crop yields, and biodiversity. Understanding geochemical processes helps scientists predict how pollutants spread through the ground or degrade over time.
2.4. Biosphere
All living organisms—from tiny microbes to large mammals—can accumulate chemicals. Fat-soluble pollutants build up in animal tissues, sometimes magnifying at higher levels of the food chain. For example, DDT once threatened bird populations by weakening eggshells. Many harmful substances degrade slowly or transform into new chemicals that still cause problems. Monitoring these pathways helps identify areas at risk, protecting wildlife and people who rely on the environment for nutrition.
3. Sources and Types of Pollutants
3.1. Natural vs. Anthropogenic
Natural processes, such as volcanic eruptions or wildfires, can emit gases and particulates. However, human activities usually release larger and more varied pollutants:
- Industrial Emissions: Factories burning fossil fuels or refining metals produce sulfur dioxide, nitrogen oxides, and particulates.
- Vehicle Exhaust: Cars, trucks, and planes emit carbon monoxide, NOₓ, hydrocarbons, and small soot particles.
- Agricultural Runoff: Fertilizers and pesticides flow from fields into rivers or groundwater.
- Urban Waste and Sewage: Landfills generate methane, and wastewater may contain pharmaceuticals and plastics.
3.2. Persistent Organic Pollutants (POPs)
POPs (e.g., polychlorinated biphenyls, dioxins, certain pesticides) remain in the environment for long periods. They can travel far from their origin via air or ocean currents, concentrating in polar regions or other remote areas. These chemicals can disrupt hormonal systems in animals and humans.
3.3. Heavy Metals
Lead, mercury, cadmium, and arsenic rank among the most hazardous metals. Industry, mining, and some consumer products release them. They do not degrade chemically and can accumulate in soils or aquatic sediments. Chronic exposure can damage the nervous system, kidneys, or other organs. Addressing metal contamination often involves removing contaminated soil, solidifying waste, or adjusting pH to immobilize metals.
3.4. Greenhouse Gases
Carbon dioxide, methane, nitrous oxide, and synthetic gases trap heat in the atmosphere. Burning coal, oil, and natural gas contributes significantly to carbon dioxide buildup. Methane comes from livestock, landfills, and natural gas operations. Nitrous oxide arises from fertilized soils and some industrial processes. These gases affect Earth’s temperature and climate patterns, leading to shifts in weather, ice melting, and sea-level rise.
4. Air Quality and Atmospheric Chemistry
4.1. Acid Rain
Sulfur dioxide and nitrogen oxides released by power plants or vehicles convert into acidic components in the atmosphere. These then fall as acid rain or snow, harming forests, lakes, and buildings. Control of sulfur emissions through scrubbers and the use of low-sulfur fuels has helped reduce acid deposition in some regions.
4.2. Smog Formation
Photochemical smog arises when sunlight triggers reactions among nitrogen oxides and volatile organic compounds, creating ground-level ozone, a strong irritant. High ozone levels damage lungs, worsen asthma, and reduce crop yields. Urban areas with heavy traffic often struggle with smog in sunny conditions. Reducing VOCs and NOₓ from vehicles and industries can improve air quality.
4.3. Particulate Matter (PM)
Fine particulate matter (PM₂.₅) and larger particles (PM₁₀) come from combustion, dust, or secondary reactions in the atmosphere. These particles can penetrate deep into the lungs or even enter the bloodstream, increasing respiratory and cardiovascular risks. Monitoring networks track PM levels, and regulators set limits, especially in dense cities.
4.4. Ozone Layer Depletion
Chlorofluorocarbons (CFCs) and related halocarbons once used in refrigerants and aerosols migrated to the stratosphere. UV radiation broke them down, releasing chlorine atoms that destroyed ozone. The Montreal Protocol restricted these substances, fostering partial recovery of the ozone layer. This example shows that global cooperation can address large-scale pollution if sustained actions continue.
5. Water Pollution and Treatment
5.1. Eutrophication
Nutrient overload from fertilizers or sewage can cause algae blooms in lakes or coastal zones. As algae die and decompose, oxygen in the water is depleted, creating “dead zones” that kill fish and other aquatic life. Reducing phosphorus and nitrogen runoff requires better farming practices and upgraded wastewater treatment.
5.2. Pathogens and Organic Wastes
Untreated sewage contains bacteria, viruses, and parasites that spread disease. Water contaminated with fecal matter can transmit illnesses like cholera or hepatitis. Biological oxygen demand (BOD) is a measure of how much oxygen microbes need to break down organic matter. High BOD can suffocate aquatic organisms. Proper sanitation and disinfection are critical for safe drinking water.
5.3. Toxic Chemical Discharges
Factories might discharge metals, solvents, or acidic effluents into rivers. Oil spills pollute oceans, poisoning marine habitats and affecting coastal economies. Treatment systems often use physical, chemical, and biological steps to remove pollutants before water enters public waterways.
5.4. Water Treatment Steps
- Coagulation/Flocculation: Added chemicals clump suspended solids, which then settle.
- Sedimentation: Sludge forms at the bottom, separated from cleaner water.
- Filtration: Sand or membrane filters remove particles.
- Disinfection: Chlorine, ozone, or UV light kills pathogens.
- Advanced Methods: Activated carbon or reverse osmosis strips out trace compounds (pesticides, pharmaceuticals).
Communities may adapt these techniques based on local water quality, cost, and regulatory frameworks.
6. Soil Contamination and Remediation
6.1. Sources of Soil Pollution
- Agricultural Chemicals: Repeated pesticide or herbicide use can accumulate in topsoil.
- Industrial Spills and Dumping: Factories may release heavy metals, petroleum byproducts, or halogenated compounds.
- Mining Activities: Exposed ore or tailings often contain toxic metals, acid-generating minerals, or sulfates.
- Landfills: Poorly sealed disposal sites leach contaminants into surrounding soil and groundwater.
6.2. Behavior of Contaminants in Soil
Soil composition (clays, organic matter, pH) affects whether chemicals bind or travel downward. Microbes can sometimes degrade organic pollutants, but heavy metals remain persistent. Understanding these interactions helps environmental scientists devise cleanup methods or land-use restrictions to protect aquifers.
6.3. Remediation Strategies
- Excavation and Removal: Contaminated soil is physically removed and treated off-site, though this can be costly.
- Bioremediation: Microorganisms break down pollutants. Adjusting oxygen, nutrients, or moisture can speed this process.
- Phytoremediation: Plants extract or stabilize toxic substances. Some hyperaccumulators concentrate metals in their tissues, which can be harvested and disposed of safely.
- Soil Washing: Uses water or chemical solvents to separate pollutants from soil particles.
- Containment/Capping: A barrier or cap reduces exposure, though contaminants remain in place.
Decisions about which technique to use depend on the type of pollutant, soil characteristics, costs, and potential risks.
7. Climate Change and Global Implications
7.1. Greenhouse Gas Effect
Solar radiation warms Earth’s surface. The planet then radiates heat outward, but certain gases trap a portion of that heat. Carbon dioxide and methane are major contributors, along with nitrous oxide and fluorinated gases. Industrialization and deforestation have upset the natural carbon cycle, causing atmospheric greenhouse gas levels to climb, which intensifies global warming.
7.2. Consequences
- Rising Temperatures: Leads to shifting climate zones, melting glaciers, and shrinking polar ice.
- Ocean Acidification: Absorption of more CO₂ increases carbonic acid in seawater, endangering corals and shellfish.
- Extreme Weather: Heatwaves, droughts, and intense storms become more frequent, threatening food security and infrastructure.
7.3. Mitigation Efforts
Countries work on lowering carbon footprints by shifting to renewable energy, improving energy efficiency, capturing carbon from power plants, or reforesting lands. International agreements aim to limit temperature increases. Meanwhile, scientists explore negative emissions technologies like direct air capture or enhanced mineral weathering. Public interest in climate solutions has led to widespread adoption of solar power, wind turbines, and electric vehicles.
8. Analytical Methods and Monitoring
8.1. Sampling Techniques
Collecting representative samples is crucial. Water may be sampled from different depths, sediment cored from various layers, and air tested at multiple locations or times. Proper containers, preservatives, and chain-of-custody records ensure the data’s reliability.
8.2. Laboratory Analysis
- Spectrometry (AAS, ICP-MS): Detect trace metals in water or soil.
- Chromatography (GC, HPLC): Separate complex mixtures of pesticides, hydrocarbons, or industrial solvents.
- Mass Spectrometry (GC-MS, LC-MS): Identify unknown compounds or confirm structures.
- Immunoassays: Use antibodies to detect specific contaminants (like certain pesticides) in field kits.
Regulators rely on standardized methods to maintain consistency across laboratories and time. Data then guides policy decisions or enforcement actions.
8.3. Remote Sensing and Modeling
Satellites observe changes in vegetation, ice cover, or pollution plumes. Remote sensors track nitrogen dioxide or aerosol densities across regions. Computer models simulate chemical transport in the atmosphere or oceans, predict smog formation under various weather conditions, or assess the fate of spilled oil. These tools help local or national agencies make contingency plans and measure progress toward emissions targets.
9. Pollution Control and Regulatory Measures
9.1. Air Pollution Abatement
- Scrubbers on power plant chimneys capture sulfur compounds.
- Catalytic Converters in car exhaust systems transform carbon monoxide and nitrogen oxides into less harmful gases.
- Fuel Switching from coal to natural gas or renewables can reduce emissions of CO₂ and particulates.
- Emissions Trading Systems set a cap on total pollution, allowing industries to trade allowances, incentivizing cuts.
9.2. Water and Wastewater Treatment
Municipal facilities treat sewage to minimize pathogens and organic load before discharge. Industrial plants often require pre-treatment to remove toxic metals or adjust pH. In rural areas, constructed wetlands or septic systems might be used, though these need careful design to avoid overflow or seepage.
9.3. Hazardous Waste Management
Hazardous wastes, such as certain chemicals, batteries, or radioactive materials, demand careful handling to prevent leaks or explosions. Secure landfills or incinerators designed with air pollution controls can neutralize or contain them. Best practices also encourage waste minimization or substitution of dangerous substances with safer options.
9.4. International Protocols
Treaties like the Stockholm Convention target persistent organic pollutants, banning or limiting chemicals such as DDT or PCBs. The Kyoto Protocol and Paris Agreement aim to curb greenhouse gas emissions. Such efforts rely on cooperation among nations, acknowledging that pollution crosses borders and affects global health.
10. Green and Sustainable Approaches
Environmental science has prompted shifts toward sustainability:
- Cleaner Production: Companies reduce raw materials, water, and energy consumption while cutting waste.
- Life Cycle Assessment (LCA): Evaluates a product’s environmental impact from raw material extraction to disposal.
- Bio-based Materials: Replacing petrochemical plastics with biodegradable alternatives from cornstarch or cellulose.
- Catalysts and Reaction Design: Innovative catalysts help industries reduce solvents or toxic intermediates. This approach lowers footprints while maintaining productivity.
- Renewable Energy: Wind, solar, hydro, and geothermal power cut fossil fuel use, diminishing greenhouse gases. Battery storage or hydrogen-based systems can help manage variability.
These concepts underscore the drive to harmonize human needs with ecological balance, aligning economic growth with care for ecosystems and the global climate.
11. Wrapping It Up
Environmental research connects chemistry, biology, physics, and social sciences to clarify how pollutants arise, spread, and can be dealt with. From acid rain reduction to the ozone layer’s partial recovery, real-world cases prove that science-based measures and public policy can limit or reverse some impacts. Still, challenges persist, notably climate shifts, plastic pollution, and emerging contaminants such as microplastics or novel chemicals.
Addressing these concerns involves a cycle of detecting and analyzing hazards, understanding their risks, and enacting control strategies. New technologies, including advanced sensors and artificial intelligence, promise faster insights into pollution sources or climate patterns. At the same time, public engagement and global collaboration remain essential for forging solutions that cross political or geographic boundaries.
Environmental research thus maintains a crucial role in guiding societies as they balance development with preserving air, water, soils, and the organisms reliant on them. The discipline’s findings translate into tools, regulations, and everyday practices that can steer humankind toward a safer, more resilient future.